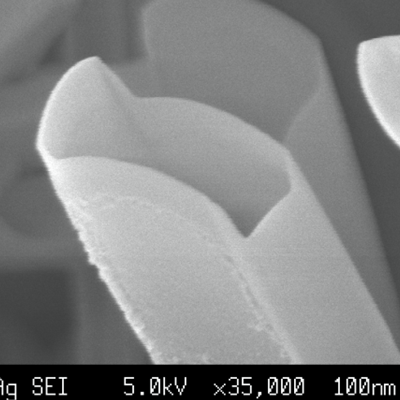
List of Recent Publication
Recent Topics
Topic 1
Diamond-Anvil Cell can pressurize liquid and gas to hundreds of thousands of atmospheric pressure due to its high airtightness. Furthermore, high-pressure and high-temperature generation is possible through combination with infrared laser heating system. We succeeded in synthesizing submicron-sized hollow crystals of rutile-type GeO2 by using supercritical oxygen as a solvent under a pressure of several GPa [1].In addition, we succeeded in synthesizing rutile-type TiO2 hollow prismatic nanocrystals used as photocatalysts by reacting water and titanium (Ti) foil instantaneously at 2-5 GPa at several thousand degrees centigrade and cooling to room temperature rapidly [2].
The high solubility of supercritical fluids under ultra-high pressure, crystal growth in a very short time (<10 ^ -3 s), and the difference in surface energy due to the crystal structure are related in synthesizing such a unique form of nanocrystalline material [1-4].In addition, we are working on the synthesis and analysis of nanocrystalline materials that are expected to add new physical properties such as the development of ferromagnetism as well as catalysts by adding trace amounts of transition metal elements such as Co [5]. Material synthesis under ultra-high pressure is a very attractive research that leads to the synthesis of a material with a completely new crystal structure, for, and function, even for known functional materials [6].
[Related papers. Students’ names from Hasegawa Lab are underlined.]
[1] K. Niwa, H. Ikegaya, M. Hasegawa, T. Ohsuna, T. Yagi, Journal of Crystal Growth 312:10 (2010) 1731-1735
[2] 長谷川正,丹羽健,セラミックス 46 (2011) 378-385
[3] 長谷川正,丹羽健,日本結晶成長学会誌 38 (2011) 4-11
[4] K. Niwa, T. Taguchi, T. Tokunaga, M. Hasegawa, Crystal Growth & Design 11:10 (2011) 4427-4432
[5] K. Niwa, H. Ikegaya, T. Taguchi, S. Muto, T. Tokunaga and M. Hasegawa, Journal of Physics: Conference Series (JPCS) 500 (2014) 022007
[6] 丹羽健,長谷川正,高圧力の科学と技術 24 (2014) 178-187
▲Page Top▲
Topic 2
Among the typical elements, nitrides of Group 14 elements have been widely studied as functional materials such as hard ceramics and catalysts. Group 14 elements generally form nitrides of A3N4(A = Si, Ge, Sn) because they are stable in tetravalent. α-type and β-type have a local structure in which nitrogen is coordinated to the group 14 element. In 1999, under high pressure of more than 10 GPa, a direct reaction between silicon (Si) and nitrogen molecule (N2) resulted in the synthesis of γ-type Si3N4 having a local structure in which N is coordinated to Si by six. Since γ-type Si3N4 has higher density and higher bulk modulus than α-type and β-type, a wide range of research has been conducted from the basics to applications in anticipation of its use as a hard material.
In 2017 we succeeded in synthesizing a pyrite type AN2 from direct reaction of group 14 elements (Si, Ge, Sn) with nitrogen molecules at 60 GPa that was predicted to exist the theoretical calculation about twenty years ago [1].Pyrite AN2 is transparent to visible light and has a higher density than α, β, and γ types. In-situ high-pressure X-ray diffraction measurement showed that the bulk modulus was higher than that of γ-type A3N4(right figure). It has been pointed out that the origin of rigidity is the bonding state of single bond nitrogen and group 14 elements existing in the crystal structure. It is expected that the origin of rigidity will be clarified by more detailed analysis in the future, and that it will be developed as a transparent rigid material that surpasses γ-type A3N4. Recently, we have succeeded in synthesizing a novel high-pressure polymorph of Sn3N4, an optical semiconductor [2].Since it has a different local structure from that of existing tin nitrides, it is expected to be applied to research toward new optoelectronic materials from the viewpoint of electronic properties.
[Related papers. Students’ names from Hasegawa Lab are underlined.]
[1] K. Niwa, H. Ogasawara, M. Hasegawa, Dalton Transactions 46 (2017) 9750-9754
[2] K. Niwa, T. Inagaki, T. Ohsuna, Z. Liu, T. Sasaki, N. A. Gaida, M. Hasegawa,CrystEngComm 22 (2020) 3531-3538
▲Page Top▲
Topic 3
In 2004, it was reported that platinum nitride was synthesized from the direct reaction of platinum and nitrogen molecules at a pressure of 40 GPa or more. Subsequent detailed crystal structure analysis and first-principles calculations revealed that this platinum nitride was PtN2. PtN2 is called platinum "per" nitride because it contains single bond nitrogen (N-N) in the crystal structure. Research has stalled due to experimentally difficult ultra-high pressure synthesis of transition metal pernitrides and polynitrides, but in 2014 we succeeded in the ultra-high pressure synthesis of other undiscovered platinum group pernitrides [1-4].And now, not only the platinum group, but also poly-nitrides of other transition metals and typical elements are being synthesized one after another, and advanced material science is being developed.
There are only several research institutes in the world, including us, with experimental facilities capable of synthesizing pernitrides. In recent years, we have been actively engaged in research on ultra-high pressure synthesis of transition metal pernitrides and succeeded in synthesizing CrN2, CoN2, and NiN2, which are pernitrides of 3d transition metal elements, besides platinum group elements [5-7].In collaboration with Soda Lab, we have been working on elucidation of the electronic structure of transition metal pernitrides by photoelectron spectroscopy using synchrotron radiation X-rays focused on micro size [8, 9].The new material synthesis method under ultra-high pressure and the analysis technology for ultra-small sample have been developed based on our original methods. Currently, we are working on further elucidating the detailed physical properties of transition metal pernitrides by improving the synthesis and evaluation techniques.
[Related papers. Students’ names from Hasegawa Lab are underlined.]
[1] K. Niwa, D. Dzivenko, K. Suzuki, R. Riedel, I. Troyan, M. Eremets and M. Hasegawa, Inorganic Chemistry 53 (2014) 697-699
[2] K. Niwa, K. Suzuki, S. Muto, K. Tatsumi, K. Soda, T. Kikegawa and M. Hasegawa, Chemistry-A European Journal 20 (2014) 1–5
[3] 丹羽健,長谷川正 高圧力の科学と技術 24 (2014) 178-187
[4] K. Niwa, T. Terabe, K. Suzuki, Y. Shirako and M. Hasegawa,
Journal of Applied Physics 119 (2016) 065901
[5] K. Niwa, T. Terabe, D. Kato, S. Takayama, M.Kato, K. Soda, M. Hasegawa, Inorganic Chemistry 56 (2017) 6410-6418
[6] K. Niwa, T. Yamamoto, T. Sasaki, M. Hasegawa, Physical Review Materials 3 (2019) 053601
[7] K. Niwa, R. Fukui, T. Terabe, T. Kawada, D. Kato, T. Sasaki, K. Soda, M. Hasegawa, European Journal of Inorganic Chemistry 33 (2019) 3753-3757
[8] K. Soda, T. Mizui, M. Komabuchi, M. Kato, T. Terabe, K. Suzuki, K. Niwa, Y. Shirako, M. Hasegawa, M. Akaogi, H. Kojitani, E. Ikenaga, Journal of the Physical Society of Japan (JPSJ) 86 (2017) 064804
[9] K. Soda, M. Komabuchi, K. Maeguchi, M. Kato, T. Terabe, K. Niwa, M. Hasegawa, Y. Ikemoto, H. Okamura, Physica B: Physics of Condensed Matter 558 (2019) 54-58
▲Page Top▲
Topic 4
Transition metal nitrides are a group of substances that show various physical properties such as superconductivity, magnetism, and hardness.
Based on the discovery of a large number of new transition metal polynitrides by the diamond anvil cell,our group has been working on the synthesis of transition metal nitrides using a multi-anvil press. Unstable azides have been used in the synthesis of transition metal nitrides using a multi-anvil press. We found that ammonium halides are a source of nitrogen.
In particular, we succeeded in nitriding tungsten, which is difficult to nitride at normal pressure, under high temperature and high pressure. Furthermore, we discovered a new tungsten nitride with MoC type structure and clarified its compression behavior[1].
In addition, a transition metal nitride was generated using ammonium halide as a nitrogen source, and a single crystal of the transition metal nitride was successfully grown[1-3].
Ammonium halides decompose at high temperatures under normal pressure, but melt at high pressures and high temperatures. It is considered that the molten ammonium halide served as a flux (auxiliary agent), and a single crystal was grown.
In addition, our group succeeded in producing tantalum nitride nanowires [2].This is the first synthesis of transition metal nitride nanowires under high pressure. Nanowires with a large surface area can be expected to improve various physical properties.
There is no example of a flux for crystal growth of such nitrides. Ammonium halide was found to be a useful nitrogen source for the synthesis of transition metal nitrides, and at the same time, an excellent flux for crystal growth of nitrides.
[Related papers. Students’ names from Hasegawa Lab are underlined.]
[1] T. Sasaki, T. Ikoma, K. Sago, Z. Liu, K. Niwa, T. Ohsuna, M. Hasegawa, Inorganic Chemistry 58 24 (2019) 16379-16386
[2] N. Gaida, T. Sasaki, Z. Liu, K. Niwa, M. Hirozawa, T. Ohsuna, M. Hasegawa, Applied Physics Letters, 116 12 (2020) 123102
[3] 生駒 鷹秀,GAIDA Nico Alexander,佐々木 拓也,丹羽 健,長谷川 正, 第48回結晶成長国内会議(JCCG-48)(2019)
▲Page Top▲
Topic 5
Phosphors are materials that emit absorbed light as light of different energy, and are also used for white LED lighting.
Our laboratory uses high-pressure synthesis and is working on the synthesis and emission control of a new high-pressure phase phosphor that is difficult to synthesize under atmospheric pressure.
We use a DIA type multi-anvil press under high pressure and synthesized Ba2Al6O11 and Sr2Al6O11
Ba2Al6O11 are possible to be synthesized under high pressure. They are the substances that we succeeded in synthesizing for the first time.
By adding small amount of Eu to these substances Sr2Al6O11 emitted blue and Ba2Al6O11 emitted blue-green light.
The difference of the color comes from the difference in the crystal structure of Ba2Al6O11and Sr2Al6O11 [1].
From the results of this research, we succeeded in synthesizing a new phosphor under high pressure and showed the usefulness of the high-pressure synthesis method in phosphor synthesis.
We are also working on controlling the emission of phosphor by synthesis under atmospheric pressure.
Y3Al5O12 is a typical substance used for phosphors, and Y3Al5O12 with Ce is also used for white LED lighting.
When a small amount of Mn is added to this Y3Al5O12, it emits red light due to tetravalent Mn.
By replacing Al with Ga and Y with Gd, our group succeeded in controlling the light emission by changing the coordination environment of the emitting Mn. Also, our group found from the detailed crystal structure analysis results that the emission is correlated with the dodecahedral site sharing the octahedral site replaced by Mn [2].
Based on the results of this research, our group succeeded in elucidating the relationship between the crystal chemistry and emission characteristics of Mn-activated phosphors.
[Related papers. Students’ names from Hasegawa Lab are underlined.]
[1] T. Sasaki, K. Niwa, M. Hasegawa, will be submitted.
[2] 安積 良太,佐々木 拓也,丹羽 健,長谷川 正, 第29回学生による材料フォーラム (2019)
▲Page Top▲
Topic 6
Understanding how nanostructures boost the physical properties of bulk materials, such as nano-polycrystalline ceramics (nanoceramics), has only been sparsely investigated. Nanoceramics offer interesting physical characteristics such as high strength and toughness, superionic conductivity, superplasticity, or high optical transparency mainly caused by the high ratio of atoms on interfaces, or crystal boundaries, to atoms in the crystals (Wollmershauser et al., Acta Mater., 2014). Probing the nature of the underlying mechanisms still faces the challenges of making bulk, fully-dense ceramics with nano-sized grains. High-pressure techniques have been proven to be a reliable tool in making fully-dense nanoceramics. High pressure suppresses self-diffusion responsible for recrystallization and grain growth at elevated temperature. Simultaneously, pressure positively affects the coordination number and the density of phases, which allows for strong intra-crystalline atomic framework bonding. Recently, several nanoceramics with unexpected and novel properties have been explored on this way, such as high optical transparency in silicate garnets (Irifune et al., Nat. Comm., 2016), cubic Si3N4 (Nishiyama et al., Sci. Rep., 2017) and triclinic aluminosilicates (Gaida et al., J. Am. Ceram. Soc., 2018; Gaida et al., J. Am. Ceram. Soc., 2020) [1-2], or a unique toughening mechanism in stishovite (Nishiyama et al., Sci. Rep., 2016).
For example, we have succeeded in the synthesis of highly transparent nanoceramics mainly consisting of triclinic and therefore birefringent crystals with enhanced mechanical properties (Gaida et al., J. Am. Ceram. Soc., 2018; Gaida et al., Int. J. Ceramic. Eng. Sci., 2020). [1,3] This was the experimental proof, that in principle, regardless of the crystal system, all solids without strong absorption in the visible-light region can potentially form transparent polycrystalline materials. The results also showed that high P-T is highly convenient to form fully-dense bulk ceramics with particle sizes from the nanometer- to micrometer scale with low particle size variation.
[Related papers. ]
[1] N. Gaida, N. Nishiyama, A. Masuno, U. Schürmann, C. Giehl, O.Beermann, H. Ohfuji, J. Bednarcik, E. Kulik, A. Holzheid, T. Irifune, L. Kienle, Journal of the American Ceramic Society 1001 (2018) 998-1003.
[2] N. Gaida, S. Gréaux, Y. Kono, H. Ohfuji, H. Kuwahara, N. Nishiyama, O. Beermann, T. Sasaki, K. Niwa, M. Hasegawa, Journal of the American Ceramic Society 00 (2020) 1-10.
[3] N. Gaida, N. Nishiyama, O. Beermann, U. Schürmann, A. Masuno, C. Giehl, K. Niwa, M. Hasegawa, S. Bhat, R. Farla, L. Kienle, International Journal of Ceramic Engineering and Science 2 (2020) 76-82.
▲Page Top▲
Staff and graduate students are working on the experiments with various research themes besides the ones above.
If you are interested in the research themes of our laboratory, please refer to the original articles from the faculty profile or ask the staff directly.
▲Page Top▲